On
Wednesday, March 28, 1979, 36 seconds after the hour of 4:00 a.m.,
several water pumps stopped working in the Unit 2 nuclear power plant
on Three Mile Island, 10 miles southeast of Harrisburg, Pennsylvania.1
Thus began the accident at Three Mile Island. In the minutes, hours,
and days that followed, a series of events -- compounded by equipment
failures, inappropriate procedures, and human errors and ignorance --
escalated into the worst crisis yet experienced by the nation's
nuclear power industry.
The accident focused
national and international attention on the nuclear facility at Three
Mile Island and raised it to a place of prominence in the minds of
hundreds of millions. For the people living in such communities as
Royalton, Goldsboro, Middletown, Hummelstown, Hershey, and Harrisburg,
the rumors, conflicting official statements, a lack of knowledge about
radiation releases, the continuing possibility of mass evacuation, and
the fear that a hydrogen bubble trapped inside a nuclear reactor might
explode were real and immediate. Later, Theodore Gross, provost of the
Capitol Campus of Pennsylvania State University located in Middletown
a few miles from TMI, would tell the Commission:
Never before have
people been asked to live with such ambiguity. The TMI accident --
an accident we cannot see or taste or smell ... is an accident that
is invisible. I think the fact that it is invisible creates a sense
of uncertainty and fright on the part of people that may well go
beyond the reality of the accident itself.2
The reality of the accident, the realization that such an accident
could actually occur, renewed and deepened the national debate over
nuclear safety and the national policy of using nuclear reactors to
generate electricity.
Three Mile Island is home to two nuclear power plants, TMI-1 and
TMI-2. Together they have a generating capacity of 1,700 megawatts,
enough electricity to supply the needs of 300,000 homes. The two
plants are owned jointly by Pennsylvania Electric Company, Jersey
Central Power & Light Company, and Metropolitan Edison Company, and
operated by Met Ed. These three companies are subsidiaries of General
Public Utilities Corporation, an electric utility holding company
headquartered in Parsippany, New Jersey.3
Each TMI plant is powered by its nuclear reactor. A reactor's function
in a commercial power plant is essentially simple -- to heat water.
The hot water, in turn, produces steam, which drives a turbine that
turns a generator to produce electricity. Nuclear reactors are a
product of high technology. In recent years, nuclear facilities of
generating capacity much larger than those of earlier years --
including TMI-1 and TMI-2 — have gone into service.4
A nuclear reactor generates heat as a result of nuclear fission, the
splitting apart of an atomic nucleus, most often that of the heavy
atom uranium. Each atom has a central core called a nucleus. The
nuclei of atoms typically contain two types of particles tightly bound
together: protons, which carry a positive charge, and neutrons, which
have no charge. When a free neutron strikes the nucleus of a uranium
atom, the nucleus splits apart. This splitting -- or fission --
produces two smaller radioactive atoms, energy, and free neutrons.
Most of the energy is immediately converted to heat. The neutrons can
strike other uranium nuclei, producing a chain reaction and continuing
the fission process. Not all free neutrons split atomic nuclei. Some,
for example, are captured by atomic nuclei. This is important, because
some elements, such as boron or cadmium, are strong absorbers of
neutrons and are used to control the rate of fission, or to shut off a
chain reaction almost instantaneously.5
Uranium fuels all nuclear reactors used commercially to generate
electricity in the United States. At TMI-2, the reactor core holds
some 100 tons of uranium. The uranium, in the form of uranium oxide,
is molded into cylindrical pellets, each about an inch tall and less
than half-an-inch wide. The pellets are stacked one atop another
inside fuel rods. These thin tubes, each about 12 feet long, are made
of Zircaloy-4, a zirconium alloy. This alloy shell -- called the
"cladding" -- transfers heat well and allows most neutrons to pass
through.6
TMI-2's reactor contained 36,816 fuel rods -- 208 in each of its 177
fuel assemblies. A fuel assembly contains not only fuel rods, but
space for cooling water to flow between the rods and tubes that may
contain control rods or instruments to measure such things as the
temperature inside the core. TMI-2's reactor has 52 tubes with
instruments and 69 with control rods.7
Control rods contain materials that are called "poisons" by the
nuclear industry because they are strong absorbers of neutrons and
shut off chain reactions. The absorbing materials in TMI-2's control
rods are 80 percent silver, 15 percent indium, and 5 percent cadmium.
When the control rods are all inserted in the core, fission is
effectively blocked, as atomic nuclei absorb neutrons so that they
cannot split other nuclei. A chain reaction is initiated by
withdrawing the control rods. By varying the number of and the length
to which the control rods are withdrawn, operators can control how
much power a plant produces. The control rods are held up by magnetic
clamps. In an emergency, the magnetic field is broken and the control
rods, responding to gravity, drop immediately into the core to halt
fission. This is called a "scram."
The nuclear reactors used in commercial power plants possess several
important safety features. They are designed so that it is impossible
for them to explode like an atomic bomb. The primary danger from
nuclear power stations is the potential for the release of radioactive
materials produced in the reactor core as the result of fission. These
materials are normally contained within the fuel rods.
Damage to the fuel rods can release radioactive material into the
reactor's cooling water and this radioactive material might be
released to the environment if the other barriers -- the reactor
coolant system and containment building barriers -- are also breached.8
A nuclear plant has three basic safety barriers, each designed to
prevent the release of radiation. The first line of protection is
the fuel rods themselves, which trap and hold radioactive
materials produced in the uranium fuel pellets.
The second barrier consists of the reactor vessel and the closed
reactor coolant system loop. The TMI-2 reactor vessel, which holds the
reactor core and its control rods, is a 40-foot high steel tank with
walls 8-½ inches thick. This tank, in turn, is surrounded by two,
separated concrete- and-steel shields, with a total thickness of up to
9-½ feet, which absorb radiation and neutrons emitted from the reactor
core. Finally, all this is set inside the containment building, a
193-foot high, reinforced-concrete structure with walls 4 feet thick.9
To supply the steam that runs the turbine, both plants at TMI rely on
a type of steam supply system called a pressurized water reactor. This
simply means that the water heated by the reactor is kept under high
pressure, normally 2,155 pounds per square inch in the TMI-2 plant.
In normal operations, it is important in a pressurized water reactor
that the water that is heated in the core remain below "saturation" --
that is, the temperature and pressure combination at which water boils
and turns to steam. In an accident, steam formation itself is not a
danger, because it too can help cool the fuel rods, although not as
effectively as the coolant water. But problems can occur if so much of
the core's coolant water boils away that the core becomes uncovered.
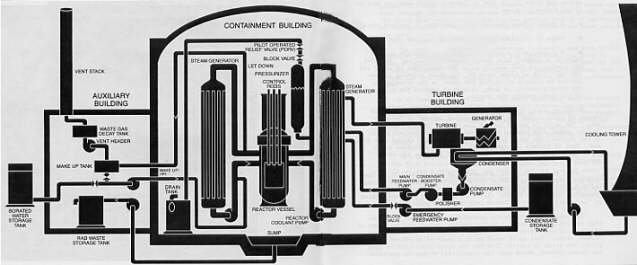
Schematic of the TMI-2 facility |
An uncovered core
may lead to two problems. First, temperature may rise to a point,
roughly 2,200°F, where a reaction of water and the cladding could
begin to damage the fuel rods and also produce hydrogen. The other
is that the temperature might rise above
the melting
point of the uranium fuel, which is about 5,200°F. Either poses a
potential danger. Damage to the zirconium cladding releases some
radioactive materials trapped inside the fuel rods into the core's
cooling water. A melting of the fuel itself could release far more
radioactive materials. If a significant portion of the
fuel should
melt, the molten fuel could melt through the reactor vessel itself
and release large quantities of radioactive materials into the
containment building. What might happen following such an event is
very complicated and depends on a number of variables such as the
specific characteristics of the materials on which a particular
containment building is constructed.10
The essential
elements of the TMI-2 system during normal operations include:
• The reactor,
with its fuel rods and control rods.
• Water, which
is heated by the fission process going on inside the fuel rods
to ultimately produce steam to run the turbine. This water, by
removing heat, also keeps the fuel rods from becoming
overheated.
• Two steam
generators, through which the heated water passes and gives up
its heat to convert cooler water in another closed system to
steam.
• A steam
turbine that drives a generator to produce electricity.
• Pumps to
circulate water through the various systems.
• A
pressurizer, a large tank that maintains the reactor water at a
pressure high enough to prevent boiling. At TMI-2 the
pressurizer tank usually holds 800 cubic feet of water and 700
cubic feet of steam above it. The steam pressure is controlled
by heating or cooling the water in the pressurizer. The steam
pressure, in turn, is used to control the pressure of the water
cooling the reactor.
Normally, water
to the TMI-2 reactor flows through a closed system of pipes called
the "reactor coolant system" or "primary loop." The water is
pushed through the reactor by four reactor coolant pumps, each
powered by a 9,000 horsepower electric motor. In the reactor, the
water picks up heat as it flows around each fuel rod. Then it
travels through 36-inch diameter, stainless steel pipes shaped
like and called "candy canes," and into the steam generators.
In the steam
generators, a transfer of heat takes place. The very hot water
from the reactor coolant system travels down through the steam
generators in a series of corrosion-resistant tubes Meanwhile,
water from another closed system -- the feedwater system or
secondary loop" -- is forced into the steam generator.
The feedwater in
the steam generators flows around the tubes that contain the hot
water from the reactor coolant system. Some of this heat is
transferred to the cooler feedwater, which boils and becomes
steam. Just as it would be in a coal- or oil-fired generating
plant, the steam is carried from the two steam generators to turn
the steam turbine, which runs the electricity-producing
generator.
The water from
the reactor coolant system, which has now lost some of its heat,
is pumped back to the reactor to pass around the fuel rods, pick
up more heat, and begin its cycle again.
The water from
the feedwater system, which has turned to steam to drive the
turbine, passes through devices called condensers. Here, the steam
is condensed back to water, and is forced back to the steam
generators again.
The condenser
water is cooled in the cooling towers. The water that cools the
condensers is also in a closed system or loop. It cools the
condensers, picks up heat, and is pumped to the cooling towers,
where it cascades along a series of steps. As it does, it releases
its heat to the outside air, creating the white vapor plumes that
drift skyward from the towers. Then the water is pumped back to
the condensers to begin its cooling process over again.
Neither the water
that cools the condensers, nor the vapor plumes that rise from the
cooling towers, nor any of the water that runs through the
feedwater system is radioactive under normal conditions. The water
that runs through the reactor coolant system is radioactive, of
course, since it has been exposed to the radioactive materials in
the core.
The turbine, the
electric generator it powers, and most of the feedwater system
piping are outside the containment building in other structures.
The steam generators, however, which must be fed by water from
both the reactor coolant and feedwater systems, are inside the
containment building with the reactor and the pressurizer tank.
A nuclear power
facility is designed with many ways to protect against system
failure. Each of its major systems has an automatic backup system
to replace it in the event of a failure. For example, in a
loss-of-coolant accident (LOCA) -- that is, an accident in which
there is a loss of the reactor's cooling water -- the Emergency
Core Cooling System (ECCS) automatically uses existing plant
equipment to ensure that cooling water covers the core.
In a LOCA, such
as occurred at TMI-2, a vital part of the ECCS is the High
Pressure Injection (HPI) pumps, which can pour about 1,000 gallons
a minute into the core to replace cooling water being lost through
a stuck-open valve, broken pipe, or other type of leak. But the
ECCS can be effective only if plant operators allow it to keep
running and functioning as designed. At Three Mile Island, they
did not. |
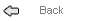 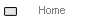 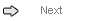
|